1. 서 론
2. 수동형채집기(passive sampler)
2.1 원리
2.2 동향
2.3 평형도달시간
3. 현장노출시간 단축 위한 연구
3.1 실행보정물질(performance reference compounds, PRCs)의 활용
3.2 물경계층(water boundary layer)의 감소
3.3 기타
4. 결 론
1. 서 론
난분해성 유기오염물질(persistent organic pollutants, POPs)은 화학적으로 매우 안정한 구조이며 화학적, 생물학적 분해에 강한 저항성을 가진다. 또한 소수성(hydrophobic)으로 인하여 환경 중에 오래 잔류하며, 생물에게 높은 농도로 축적되고 먹이사슬을 통한 생물확대(biomagnification)로 인간에게까지 영향을 미칠 수 있다(UNEP, 2001; UNEP, 2004). 이러한 오염물질은 해양환경 중에서 입자(0.45 um 이상), 콜로이드(colloid) 또는 용존유기탄소(dissolved organic carbon, DOC)에 흡착되어 있거나, 물속에서 자유롭게 용존 되어있는 자유용존상(freely dissolved phase)으로 존재한다(ITRC, 2011; USEPA, 2012; Booij et al., 2016). 이 중 해양생물이 실제로 이용할 수 있는 형태는 해수나 공극수 내에서의 자유용존상으로, 해수 및 퇴적물의 생물학적 영향 및 위해도를 보다 정확히 평가하기 위해서는 자유용존상 농도(freely dissolved and bioavailable concentration, Cfree)를 파악하는 것이 매우 중요하다(Di Toro et al., 1991; Perron et al., 2013a).
일반적으로 해수에서 난분해성 유기오염물질의 농도는 pg/L ~ ng/L 수준으로 매우 낮으며, 이를 측정하기 위하여 엄청난 양의 시료를 채취한 뒤(grab sampling), 여과, 액-액 추출 혹은 고정상 추출(solid phase extraction, SPE)하여 얻은 추출액을 분석함으로써 용존상 농도를 측정하였다(Booij et al., 2016). 그러나 이러한 방법은 전처리 과정이 복잡하여 시간이 오래 소요되며 분석되더라도 종종 검출한계 이하 값으로 측정된다(Smedes, 1994; Bao et al., 2012). 또한 공극수에서의 용존상 농도(dissolved concentration in porewater, Cd)는 해수에서와 같은 직접 추출분석법 이외에 퇴적물에서의 오염물질의 농도(concentration in sediment, Cs)와 퇴적물 내 유기탄소와 공극수 사이에서의 평형분배(sediment-water partitioning coefficient, Kd)로부터 구할 수 있으며(식 (1), (2))(Di Toro et al., 1991), 블랙카본(black carbon)이나 수트(soot)과 같은 유기탄소에 강하게 흡착되어 실제 생물에 이용되어질 수 없는 조건에 대해서도(Gustafsson et al., 1996; Jonker and Koelmans, 2002; Lohmann et al., 2005) 보정해 줄 수 있는 모델식이 개선되어 왔다(식 (3))(Accardi-Dey and Gschwend, 2002; Martinez et al., 2013; Apell and Gschwend, 2014). 하지만 이러한 모델 식으로부터 예측한 독성치 역시 실제 생물독성실험결과와 일치하지 않는 사례가 종종 보고되었다(McDonough et al., 2010).
$${\mathrm C}_{\mathrm d}=\frac{{\mathrm C}_{\mathrm s}}{{\mathrm K}_{\mathrm d}}$$ | (1) |
$${\mathrm K}_{\mathrm d}={\mathrm f}_{\mathrm{OC}}{\mathrm K}_{\mathrm{OC}}$$ | (2) |
$${\mathrm K}_{\mathrm d}={\mathrm f}_{\mathrm{OC}}{\mathrm K}_{\mathrm{OC}}+{\mathrm f}_{\mathrm{BC}}{\mathrm K}_{\mathrm{BC}}\mathrm C_{\mathrm{free}}^{\mathrm n-1}$$ | (3) |
Cd: Dissolved concentration in porewater
Cs: Concentration in sediment
Kd: Sediment-water partitioning coefficient
fOC: Fraction of organic carbon in sediment (=mOC/ms)
KOC: Organic carbon-water partitioning coefficient (=COC/Cd)
fBC: Fraction of black carbon in sediment (=mBC/ms)
KBC: Black carbon-water partitioning coefficient (=CBC/Cd)
1990년대 이후부터 위와 같은 복잡한 절차를 줄이고 보다 정확한 생물학적 영향을 파악하기 위해 자유용존상 농도 측정법 개발의 필요성이 제기되었고, 이를 위해 수동형채집기(passive sampler)가 고안되었다. 수동형채집기란 생물의 지방과 유사한 유기 물질이 분배평형 원리에 따라 유기오염물질을 흡수하는 성질을 이용한 생체모방형 장치로서, 용존유기탄소와 같은 거대분자는 흡수하지 않고 생물이 실제로 이용할 수 있는 자유용존상 유기오염물질만을 흡수한다. 따라서 회수되어진 수동형채집기로부터의 자유용존상 농도 측정은 기존의 분석법과 달리 화학분석이 용이하며, 낮은 검출한계로 인해 pg/L 수준의 매우 낮은 농도까지 검출이 가능하다(Mayer et al., 2000; Mills et al., 2011; Sacks and Lohmann, 2012; Bao et al., 2012; Perron et al., 2013b; Moschet et al., 2014). 무엇보다도 수동형채집기를 이용해 얻은 자유용존상 농도는 모든 존재형태(입자태+용존태)를 합한 총 농도(total concentration, Ctotal)보다 생물농축 및 독성 결과에 대해 보다 정확한 정보를 제공해 준다(van del Wal et al., 2004; You et al., 2006; Lu et al., 2011; Muijs and Jonker, 2012; Joyce et al., 2015).
이러한 장점으로 수동형채집기를 활용한 국내에서의 연구는 대기분야에서 꾸준히 수행되고 있지만(Choi et al., 2009; Heo and Lee, 2014; Kim et al., 2019), 육상 및 해양환경에서의 경우 긴 평형도달시간에 따른 수동형채집기에 생물부착(biofouling) 및 수동형채집기의 현장 적용시 어려움으로 인해 보고된 연구 사례는 매우 제한적이다(An et al., 2014; Kim et al., 2014; Kim et al., 2015). 반면 유럽이나 미국에서는 수동형채집기의 장시간 현장 적용에 대한 문제점을 해결하기 위한 연구가 꾸준히 수행되어 왔으며, 최근에는 매질별 현장 적용에 대한 가이드라인도 제시하고 있다(USEPA, 2012; Miège et al., 2015; USEPA, 2017).
따라서 1장은 난분해성 유기오염물질을 대상으로 하는 수동형채집기의 종류 및 특징, 국외 해양환경에서의 적용 사례에 대한 고찰 등을 다루었으며(Lee at al., in press), 2장인 본 논문에서는 난분해성 유기오염물질을 대상으로 하는 수동형채집기의 동향을 파악하고, 수동형채집기별 평형에 도달하는데 소요되는 시간, 그리고 현장노출 시간 단축을 위한 다양한 연구사례를 수집하고 검토함으로써 국내 해양환경에서의 수동형채집기의 적용을 활성화하고자 한다.
2. 수동형채집기(passive sampler)
2.1 원리
수동형채집기의 원리는 Fig. 1에 나타내었으며, a는 수동형채집기가 물에서 자유용존상으로 존재하는 물질을 흡수하는 모식도이며, b는 시간에 따른 수동형채집기에서의 자유용존상 농도를 나타낸 것이다. 수동형채집기를 해수나 퇴적물의 공극수와 같은 특정 매질에 노출하였을 때, 초기에는 확산(diffusion)에 의해 물에서의 자유용존상 난분해성 유기오염물질이 수동형채집기로 빠른 속도로 흡수된다(kinetic region). 이 후 일정시간이 지나면 수동형채집기와 매질 간 자유용존상이 평형에 도달하면서 농도가 일정해진다(equilibrium region) (Vrana et al., 2005; USEPA, 2017). 따라서 평형에 도달하였을 때 물에서의 난분해성 유기오염물질의 자유용존상 농도(Cfree)는 수동형채집기에서의 농도(Cp∞)와 수동형채집기/물간의 분배계수(passive sampler-water partition coefficient, Kpw)를 이용하여 구할 수 있으며(식 (4)), 수동형채집기의 Kpw는 사전에 실험실에서 실험을 통해 얻어진 값으로 사용한다(식 (5)). 평형에 도달하기 이전에는 특정 시간(t)일 때 수동형채집기에서의 난분해성 유기오염물질의 농도(Cpt)와 실행보정물질(performance reference compounds, PRCs)로부터 얻어진 속도교환상수 ke 값으로부터 평형에 도달하였을 때의 수동형채집기에서의 농도(Cp∞)(식 (6))와 물에서의 자유용존상 농도(Cfree)(식 (4))를 계산할 수 있다.
$${\mathrm C}_{\mathrm{free}}=\frac{\mathrm C_{\mathrm p}^\infty}{{\mathrm K}_{\mathrm{pw}}}$$ | (4) |
$${\mathrm K}_{\mathrm{pw}}=\frac{\mathrm C_{\mathrm p}^\infty}{\mathrm K_{\mathrm w}^\infty}$$ | (5) |
$$\mathrm C_{\mathrm p}^{\mathrm t}=\mathrm C_{\mathrm p}^\infty\times(1-\mathrm e^{-{\mathrm k}_{\mathrm e}\mathrm t\;})$$ | (6) |
Cfree: Freely dissolved concentration (ng/L) of target compound in water
Cp∞: Concentration (ng/L) of target compound in passive sampler at equilibrium
Kpw: Passive sampler-water partitioning coefficient
Cw∞: Concentration (ng/L) of target compound in water at equilibrium
Cpt: Concentration (ng/L) of target compound in passive sampler at time t
ke: Exchange rate coefficient
한편 수동형채집기의 분배계수(Kpw)는 온도 및 염분과 같은 환경요인에 영향을 받는다. 저밀도폴리에틸렌(low density polyethylene, LDPE)와 반투과성막 장치(semi-permeable membrane devices, SPMDs)의 분배계수의 경우 물의 온도가 2°C일 때 LDPE의 분배계수는 30°C보다 약 2배 높게 나타났지만, SPMDs의 분배계수는 온도에 대한 영향은 관찰되지 않았다(Booij et al., 2003). silicone rubber (SR)의 경우 온도가 감소함에 따라 분배계수가 증가하는 패턴을 보였으며, 화합물의 소수성이 낮을수록 온도에 따른 분배계수 변화가 더욱 뚜렷하게 증가하는 것으로 나타났다. 반면 염분은 염도가 증가함에 따라 분배계수가 증가하는 경향을 나타내었으나 통계적으로 유의한 차이를 나타내지는 않았다(Jonker et al., 2015). 따라서 실험실에서 얻어진 분배계수는 현장에서의 온도 및 염분에 따른 보정이 필요하며(Rantalainen et al., 2000; Booij et al., 2003; Adams et al., 2007; Reitsma et al., 2013), 이에 대한 보정에는 식 (7)~(9)를 이용할 수 있다(Lohmann, 2012; Jonker et al., 2015).
$${\mathrm K}_{\mathrm{PE}-\mathrm w}\;(\mathrm T)={\mathrm K}_{\mathrm{PE}-\mathrm w}\;(298)\mathrm e^{(\triangle{\mathrm H}_{\mathrm{PE}-\mathrm w}/\mathrm R)\frac1{298}-\frac1{\mathrm T}}$$ | (7) |
$$\mathrm C_{\mathrm w}^{\mathrm{salt}}={\mathrm C}_{\mathrm w}10^{-{\mathrm K}_{\mathrm S}\;\lbrack\mathrm{salt}\rbrack}$$ | (8) |
KPE-w (T): PE-water partitioning coefficient at temperature T (K)
KPE-w (298): PE-water partitioning coefficient at temperature 298 K
△HPE-w: Enthalpy of PE-water partitioning
R: Universal gas constant (8.3143 J/mol/K)
Ks: Salting out constant (M-1)
[salt]: Molar ionic strength (M) of the aqueous solution
$$\log\;{\mathrm K}_{\mathrm{sr}-\mathrm w}\;(\mathrm T,\;\mathrm S)=\log\;K_{sr-w}\;(20,0)+\gamma\;(T-20)+0.0033S$$ | (9) |
Ksr-w (T): SR-water partitioning coefficient at temperature T (℃) and salinity S (g/L)
γ: (Native) compound-specific temperature dependency factor
2.2 동향
난분해성 유기오염물질을 대상으로 개발된 초기 수동형채집기로는 지질과 유사한 트리올레인(triolein)을 LDPE 이중막 사이에 채운 SPMDs이다(Huckins et al., 1990). 해수 중 용존상 유기오염물질은 5-10 Å 크기의 공극을 가진 LDPE를 통과한 후 트리올레인에 흡수되며, 트리올레인에 흡수된 오염물질의 양과 흡수속도(Sampling rate, Rs)로 부터 자유용존상 농도를 계산하였다. Fig. 2는 대기를 제외한 육상 및 해양 환경에 적용된 수동형채집기의 종류에 따른 논문 게재 편수를 나타낸 그래프이다. 1995년 SPMDs가 처음으로 육상 및 해양 환경에 적용되었으나(Fig. 3a)(Prest et al., 1995; Booij et al., 2002b), 현장 적용 시 LDPE가 찢어질 경우 트리올레인의 회수가 어렵고 단일 재질의 LDPE가 SPMDs와 유사한 흡수 능력을 가진 것을 확인함으로써(Lefkovitz et al., 1996) SPMDs의 활용도는 침체되면서 LDPE의 사용이 급격히 증가되기 시작하였다(Muller et al., 2001; Adams et al., 2007; Bao et al., 2012; Borrelli et al., 2018). 현재는 SPMDs와 유사한 흡수 능력을 가진 값싼 단일 재질의 polyoxymethylene (POM) (Jonker and Koelmans, 2001; Cornelissen et al., 2008a; Hawthorne et al., 2011), SR (Smedes, 2007; Prokes et al., 2012; Yates et al., 2013)등을 다양한 틀에 장착시켜 현장에 적용하고 있다(Fig. 3b)(Gschwend et al., 2011; Liu et al., 2013; Perron et al., 2013a; Allan et al., 2013; Bao et al., 2014). 특히 SR은 LDPE보다 logKow가 넓은 범위의 화합물을 검출할 수 있다고 보고된 바 있으며(Allan et al., 2013), 신규살충제나 인공향료, 유기인계 난연제 등 친수성을 띄는 신규 유기오염물질 분석에 점점 더 많이 활용되어지고 있다(Ahrens et al., 2015; Martin et al., 2016; Pintado-Herrera et al., 2016). 한편 solid phase microextraction (SPME) fiber는 ㎛ 단위 직경의 유리관에 오염물질을 흡수하는 polydimethylsiloxane (PDMS)가 얇게 코팅된 바늘 형태로(Fig. 3c), 쉽게 깨지거나 부피가 적어 검출한계가 높은 단점이 있다. 그러나 현장에 적용된 SPME는 전처리없이 분석장비에 곧바로 주입하므로써 기기분석이 가능하고 생물 체내에 이식하여 직접적인 생물학적 영향을 파악할 수 있어 높은 활용도를 가진다(Zeng et al., 2004; Ossiander et al., 2008; Zhang et al., 2010; Witt et al., 2013; Maruya et al., 2015).
2.3 평형도달시간
수동형채집기의 평형모델을 이용해 해양환경에서의 자유용존상 농도를 측정하기 위해서는 자유용존상의 오염물질이 수동형채집기와 매질 사이에 평형에 도달할 때까지 수동형채집기를 현장에 설치하여야 한다. Apell et al.(2016a)은 LDPE를 해수 및 퇴적물에서 노출하였을 때 다환방향족탄화수소(polycyclic aromatic hydrocarbons, PAHs)가 95% 이상 평형에 도달하기까지 걸리는 시간을 “timescale” 개념을 이용하여 계산하였다. 그 결과 해수 및 퇴적물에서 소수성이 작은 나프탈렌(물-옥탄올 분배계수, logKow=3.4)의 평형도달시간은 각각 0.1 일과 15 일이지만, 소수성이 큰 벤조(a)피렌(logKow=6.3)의 경우 174 일과 104.66 일로 예측되었다.
수동형채집기의 평형도달시간은 재질의 종류에 따라 다르나, 일반적으로 재질의 두께가 두꺼울수록, 분석대상물질의 소수성이 큰 물질(logKow>6) 일수록 오래 걸린다(Table 1). 예를 들어 POM(두께 55 ㎛)과 PDMS(두께 28 ㎛)로 코팅된 SPME를 해수에 노출하였을 때 PAHs는 약 한 달 만에 평형에 도달하였으나, 보다 두꺼운 POM(두께 500 ㎛)과 LDPE(두께 100 ㎛)의 경우 119일이 지나도 평형에 도달하지 못하였다(Cornelissen et al., 2008b). 또한 McDonough et al.(2018)에 의하면 LDPE(두께 50 ㎛)를 20~70일간 표층 해수에 노출하였을 때, 12종의 organophosphate esters 중에서 logKow가 가장 낮은 2종만이 PE에 흡수 및 평형에 도달한 것으로 예측되었다. 또한 대부분의 수동형채집기는 해수나 퇴적물에서 대략 1-3달 정도 노출하지만, SPME를 제외한 나머지 재질들의 경우 logKow가 6 이상인 화합물에 대해서는 평형에 도달하지 않는 경향을 보인다.
만일 평형에 도달하는 시간이 길어져 수동형채집기가 현장에 장기간 노출될 경우, 수동형채집기 표면에 생물부착(biofouling)으로 인해 오염물질의 흡수속도가 감소되거나(Prest et al., 1992; Richardson et al., 2002) 수동형채집기의 유실 또는 흡수된 분석대상물질들이 분해될 수 있는 위험이 있다(Liu et al., 2014).
Table 1. Field deployment time for diverse passive sampler in marine environment
Matrix | Material | Thickness (㎛) | Analyte | logKow | Level of Equilibrium | Deployment (days) | Reference |
Seawater | LDPEa | 25 | 7DDxe, 20PCBsf | 5.5~8.2 | · For DDx, >70% equilibrium only for p,p’-DDT/DDD · For PCBs, 90% equilibrium only for PCB28 and 52 | 44 | Fernandez et al.(2014) |
25.4 | 12PBDEsg | 4.3~8.4 | Equilibrium for analytes (logKow<5.3) | 30 | Sacks and Lohmann.(2012) | ||
50 | 12OPEsh, 12PBDEs | 1.4~8.4 | Equilibrium only for analytes (logKow 1.4 and 2.6) | 20~70 | McDonough et al.(2018) | ||
100 | 14PAHs | 3.4~6.9 | No equilibrium | >119 | Cornelissen et al.(2008b) | ||
POMb | 17 | 12PAHs, 6PCBs | 4.5~7.9 | Equilibrium except for analytes (logKow>7.2) | 60 | Cornelissen et al.(2009) | |
55 | 14PAHs | 3.4~6.9 | Equilibrium | 23-63 | Cornelissen et al.(2008b) | ||
76 | 7DDx, 20PCBs | 5.5~8.2 | · For DDx, >50% equilibrium · For PCBs, >40% equilibrium | 44 | Fernandez et al.(2014) | ||
500 | 14PAHs | 3.4~6.9 | No equilibrium | >119 | Cornelissen et al.(2008b) | ||
SPMEc (PDMS) | 28.5 | 14PAHs | 3.4~6.9 | Equilibrium | 23~63 | Cornelissen et al.(2008b) | |
100 | 7PCBs, 4DDx | 5.6~6.9 | Equilibrium for p,p'-DDE (the others: ND) | 18~23 | Zeng et al.(2004) | ||
SRd | no data | 16PAHs, 14PCBs | 2.8~7.6 | >80% equilibrium for analytes (logKow 2.8~4.2) | 60 | Monteyne et al.(2013) | |
Sediment | LDPE | 10 | 6DDx | 6.1~6.9 | 70% equilibrium for analytes (logKow 6.9) | 30 | Borrelli et al.(2018) |
25 | 6DDx | 6.1~6.9 | 40% equilibrium for analytes (logKow 6.9) | 30 | Borrelli et al.(2018) | ||
25 | 7DDx, 20PCBs | 5.5~8.2 | · For DDx, >80% equilibrium only for p,p’-DDT/DDD · For PCBs, >60% equilibrium for only for PCB28 and 52 | 44 | Fernandez et al.(2014) | ||
POM | 76 | 7DDx, 20PCBs | 5.5~8.2 | >50% equilibrium | 44 | Fernandez et al.(2014) | |
SPME (PDMS) | 10 | 11PAHs, 7PCBs | 4.5~7.9 | Equilibirum | 70 | Witt et al.(2013) | |
30 | 11PAHs, 7PCBs | 4.5~7.9 | Equilibirum | 70 | Witt et al.(2013) | ||
SR | no data | 30PAHs | 3.4~7.6 | Equilibrium | 20 | Yates et al.(2013) |
3. 현장노출시간 단축 위한 연구
3.1 실행보정물질(performance reference compounds, PRCs)의 활용
현장노출시간을 단축시킬 수 있는 가장 일반적인 방법은 분석대상물질이 수동형채집에 평형상태에 도달하지 않더라도 실행보정물질(performance reference compounds, PRCs)을 이용해 평형상태의 자유용존상 농도로 보정하는 것이다(Fernandez et al., 2012; Apell and Gschwend, 2014; Xu et al., 2018). 실행보정물질은 분석대상물질의 물리화학적 성질(분자 부피, 확산, 분배계수 등)과 유사한 화합물을 선택해야 하므로 주로 분석대상물질의 안정성 동위원소(13C, 2H 등)로 치환된 화합물을 사용한다. 단 실험실에서 정도관리를 위해 사용하는 내부표준물질과는 반드시 다른 물질이어야 한다(USEPA, 2017).
실행보정물질 개념은 Huckins et al.(2002)이 처음 도입했으며, 방법은 다음과 같다. 먼저 수동형채집기를 높은 농도의 실행보정물질 용매에 담근 후 교반시켜 충분한 양의 실행보정물질을 흡수시킨다. 이 때 methanol:water (80:20, v/v) 혼합용매를 사용하게 되면, 메탄올이 수동형채집기의 재질 내부를 팽창시켜 실행보정물질 흡수를 촉진시키므로 흡수시간을 단축시킬 수 있다. 증류수에 희석된 실행보정물질을 LDPE에 흡수시킬 경우 PAHs와 PCBs를 대상으로 최소 30일이 소요되지만, methanol:water (80:20, v/v) 혼합용매에서는 약 7일 만에 평형에 도달하는 것을 확인하였다(Booij et al., 2002a). 이러한 용매를 이용한 실행보정물질 흡수방법은 LDPE, POM, SR에 대해서도 적용가능하다(Perron et al., 2013a; Monteyne et al., 2013).
충분한 양의 실행보정물질이 흡수된 수동형채집기를 현장에 노출하였을 때, 수동형채집기 내의 실행보정물질은 환경 매질로 빠져 나가며 이와 유사한 속도로 분석대상물질이 흡수된다(Rusina et al., 2010; Yates et al., 2013). 따라서 분석대상물질의 속도교환계수(exchange rate coefficient, ke)는 실행보정물질의 ke값과 동일하다고 가정하였을 때, 수동형채집기에서의 실행보정물질의 초기 농도(C0p,PRC)와 시간 t에서의 농도(Ctp,PRC)의 회귀곡선으로부터 ke를 구할 수 있으며(식 (10))(Adams et al., 2007), 식 (6)과 (4)로부터 평형상태에서의 자유용존상 농도를 예측할 수 있다.
$${\mathrm K}_{\mathrm e}=\ln\;(\frac{C_{\mathit p}^{\mathit0}\mathit,PRC}{C_{\mathit p}^{\mathit t}\mathit,PRC})\times t^{-1}$$ | (10) |
C0p,PRC: Concentration (ng/L) of PRC in passive sampler at time 0
Ctp,PRC: Concentration (ng/L) of PRC in passive sampler at time t
실행보정물질을 사전에 흡수시킨 수동형채집기는 수동형채집기 내 실행보정물질이 최소 10% 이상 빠져나가는 데 걸리는 시간만 현장에 노출하여도 자유용존상 농도를 계산할 수 있다. 그러나 기기오차를 5%라 가정할 때, 최소 17%의 실행보정물질이 손실되어야 실행보정물질로 보정된 값이 실측값과 2배 내외로만 차이나며, 12% 이하로 손실될 경우 실측값과 약 4배 가까이 차이난다(Apell and Gschwend, 2014). 따라서 수동형채집기 내 실행보정물질이 최소 20% 이상 감소하는 데 필요한 시간만큼 현장에 노출하여야 실행보정물질로부터 보정된 분석대상물질의 값을 신뢰할 수 있다(Apell and Gschwend, 2014; Joyce et al., 2015; Apell et al., 2016b).
3.2 물경계층(water boundary layer)의 감소
3.2.1 해수
수동형채집기를 해수에 노출할 경우, 해수에서의 오염물질은 수동형 채집기 재질 표면에 존재하는 biofilm을 통과하여 내부로 확산된다. 따라서 물질전달에 대한 전체 저항(Io)은 biofouling이 존재하지 않을 경우 수동형채집기의 재질 내부에서의 저항(1/kmKmw) 그리고 재질 표면에 존재하는 물경계층(water boundary layer, WBL)에서의 저항(1/kw)에 의해 제어된다(식 (11))(Booij et al., 2003; Huckins et al., 2006).
$${\mathrm I}_{\mathrm o}=\frac1{{\mathrm k}_{\mathrm o}}=\frac1{{\mathrm k}_{\mathrm w}}+\frac1{{\mathrm k}_{\mathrm m}\;{\mathrm K}_{\mathrm{mw}}}$$ | (11) |
Io: Resistance to overall mass transfer
ko: Overall mass transfer
kw: Mass transfer coefficients for the water boundary layer
Kmw: Mass transfer coefficients for the membrane
일반적으로 logKow가 낮은 화합물의 경우 물경계층의 저항보다 재질 내부의 저항에 의해 흡수속도가 제어된다. 그러나 이러한 화합물은 대부분 현장에서 짧은 노출 기간 내에 평형에 도달하므로 재질 내부의 저항은 흡수속도에 크게 영향을 미치지 않는다(Anderson et al., 2008; Lohmann et al., 2012a). 반면 logKow가 높은 화합물은 물질전달, 즉 흡수속도에 영향을 주는 주요 요인이 재질 내부에서 물경계층으로 전환된다. 그 기준은 노출되는 환경의 유체역학 조건, 수동형채집기 재질의 확산계수 및 두께에 따라 다르며 SPMD, LDPE의 경우 logKow를 기준으로 4.5~5이며, SR는 3이다(Huckins et al., 1999; Anderson et al., 2008; Rusina et al., 2010; Booij et al., 2016).
logKow가 높은 소수성 화합물을 대상으로 흡수속도를 높이기 위해서는 수동형채집기 주변의 물에 난류를 형성시키거나 유속을 높임으로써 물경계층에 의한 저항을 감소시킬 수 있다(Booij et al., 2003; Huckins et al., 2006). 수동형채집기 주변에 인위적으로 난류를 형성하게 되면 물경계층이 감소함으로써 단시간에 많은 양의 오염물질을 흡수할 수 있으며, 실행보정물질을 이용할 경우 보다 정확한 보정이 가능하다(Vrana and Schüürmann, 2002; Booij et al., 2003) (Fig. 4). 난류 형성을 활용한 연구로는 전기드릴이나 진동모터를 수동형채집기에 장착 후 작동시켜 현장에 노출하거나(Qin et al., 2009; Jalalizadeh and Ghosh, 2016), 선박 뒤에 수동형채집기를 설치한 후 빠른 속도로 예인한 사례가 있다(Booij et al., 2007; Allan et al., 2011; Lohmann et al., 2012b; Vrana et al., 2015). Lohmann(2012)는 두께가 51 ㎛인 LDPE를 매우 빠른 난류에 노출시켜 물경계층에 의한 저항이 무시될 수 있는 조건일 경우, 대부분의 난분해성 유기오염물질이 24 시간 만에 평형에 도달할 것으로 예측하였다.
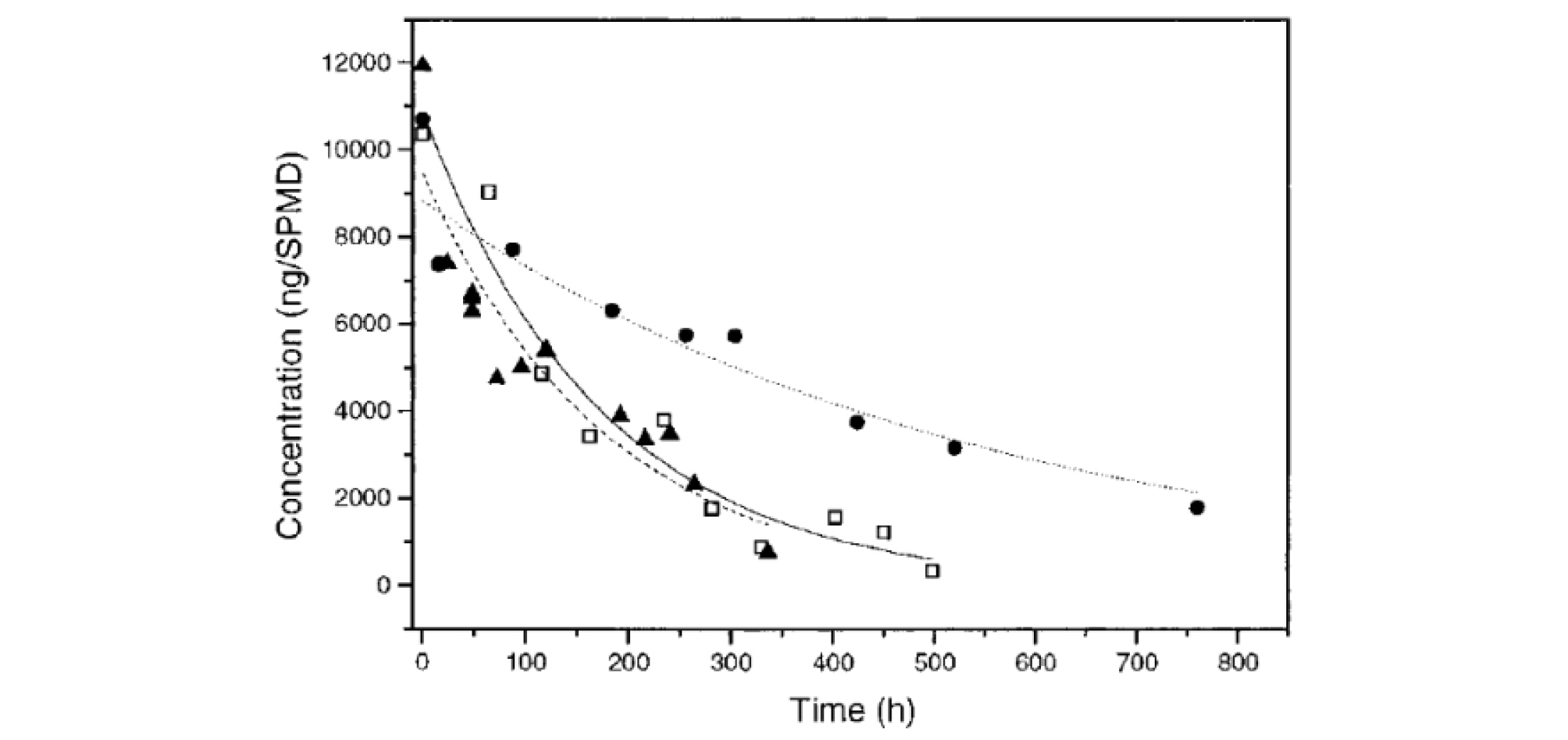
Fig. 4.
Release of [2H10]anthracene from SPMDs exposed at different linear flow velocities: 0.06 cm/s (●, dotted lines), 0.28 cm/s (▲, dashed lines), and 1.14 cm/s (□, solid lines) (Adapted from Vrana and Schüürmann, 2002, Environ. Sci. Technol., 36: 290-296, with permission of American Chemical Society).
3.2.2 퇴적물
해수와 달리 퇴적물은 공극수와의 확산이 느려 실행보정물질을 적용하더라도 신뢰성 높은 값을 산출하기 위해서는 오랜 노출시간이 요구된다. 따라서 퇴적물의 경우 수동형채집기를 현장에 직접 노출(in situ)하지 않고, 현장시료를 채취해 실험실에서 시료를 슬러리(slurry) 상태로 만들어 수동형채집기와 혼합 후 교반하여 평형도달시간을 단축시키는 방식(ex situ)을 개발하였다(Allan et al., 2012; Witt et al., 2013; Mayer et al., 2014; Endo et al., 2017). 두께 75 ㎛인 POM을 ex situ 방식으로 퇴적물 슬러리에 넣고 교반하였을 때 퇴적물 내 PAHs가 28일 만에 POM과 평형에 도달하였으며(Hawthorne et al., 2011), 심지어 두께 2~8 ㎛인 PDMS가 코팅된 유리용기에 노출하였을 때 logKow가 5~8사이인 PCBs가 약 2주 만에 평형에 도달하였다(Jahnke et al., 2012).
Jonker et al.(2018)는 11개의 실험실에서 14개의 수동형채집기를 ex situ 방법으로 동일한 퇴적물 공극수에서의 자유용존상 농도를 측정하여 비교하였다. 그 결과 각 실험실에서 산출한 자유용존상 농도 값은 약 10배 정도 차이를 나타냈지만, 제공되어진 표준화되어진 방법(standardized operational procedure, SOP)으로 분석하였을 때는 값의 차이가 절반으로 감소하였다. 나머지 편차에 대한 주요 요인은 수동형채집기 자체의 문제가 아닌 퇴적물 균질화 및 분석 오차로 확인되었다. 또한 Borrelli et al.(2018)에 의하면 PE를 이용해 ex situ 방식으로부터 측정된 자유용존상 농도는 퇴적물의 유기탄소나 블랙카본으로 보정된 분배평형모델 식으로부터 얻어진 농도보다 현장에 직접 노출한 PE로부터 계산되어진 값과 유사하게 나타났다(Fig. 5).
Ex situ 방식에서 주의해야 할 점은 시료 내 오염물질이 수동형채집기에 흡수되더라도, 전체 시료에서의 오염물질의 양에는 영향을 미치지 않아야 한다(non-depletion). 만약 이러한 현상(depletion)이 발생하면 계산되어진 자유용존상 농도는 실제 환경에서의 값보다 낮아지게 된다(underestimated). 따라서 수동형채집기의 노출 후 시료의 오염물질의 농도 감소 허용 범위는 최대 5% 이하로 유지되어야 하며, 이러한 조건을 성립하기 위해서는 수동형채집기와 퇴적물 내 유기탄소의 무게 비가 5:100 이하가 되도록 설정하여야 한다(Mayer et al., 2014; Ghosh et al., 2014; Jonker et al., 2018). 또한 노출시간동안 시료 내의 오염물질의 미생물 분해를 방지하기 위해 NaN3나 HgCl2를 25~200 mg/L 첨가해야 한다(Ghosh et al., 2014). 그러나 ex situ 방식은 해류, 지하수 유입, 생물교란 작용 등과 같은 현장조건을 반영할 수 없는 단점이 있으므로, 퇴적물과 물 사이의 플럭스나 생물학적 영향을 정확히 파악하기 위해서는 수동형채집기를 현장에 직접 노출(in situ)하는 것이 더 바람직하다(Ghosh et al., 2014; Apell and Gschwend, 2016b).
3.3 기타
이 외에도 수동형채집기의 흡수효율을 높이기 위해서는 수동형채집기의 형태를 튜브 형 보다는 평판 또는 벨트 형으로 제작함으로써 해수와의 표면적을 넓히거나, 현장 유속의 흐름과 수평하게 설치하는 것을 추천한다(Vrana et al., 2005; Lao et al., 2019). 또 최근에는 PMMA (poly (methyl methacrylate))라는 소재를 특정 유기용매에 노출함으로써 물성을 변화시켜(swelling) 흡수효율을 높이거나(Lao et al., 2016), 소혈청알부민(bovine serum albumin, BSA)을 이용해 물경계층에서의 물질 전달을 촉진시켜 평형도달시간을 단축한 연구사례 등이 있다(Endo et al., 2017).
수동형채집기는 특히 해수에서 장시간 현장 노출할 경우 재질 표면에 부착된 생물 을 제거하기란 매우 번거롭다. Fig. 6은 보호 장치 여부에 따라 해수에 4개월간 노출한 수동형채집기의 전후의 모습이다. a와 b는 LDPE를 보호 장치 없이 해수에 노출한 전과 후의 사진으로, 4개월이 지난 LDPE에서는 부착생물을 물리적으로 제거하는 데 한계가 있었다. 반면 c와 d는 스테인레스 소재의 보호구를 장착한 PMMA의 노출 전후 사례로, 노출 후 PMMA의 보호구 표면에는 부착생물이 존재하였으나 재질 표면에서는 관찰되지 않았으며 붙어있는 이물질 역시 손쉽게 제거할 수 있었다. 따라서 최소 한 달 이상 현장에 적용할 경우, 구리나 스테인레스 소재의 케이스를 활용하는 것을 추천한다(Witt et al., 2013; Ghosh et al., 2014).
4. 결 론
수동형채집기는 해수나 퇴적물 내 공극수에서의 자유용존상 농도를 쉽고 보다 정확히 예측할 수 있는 유망한 방법 중 하나이다. 그러나 현장노출시 긴 평형도달 시간으로 생물부착의 생성, 분석대상물질의 흡수가 저해되는 한계점이 있다. 수동형채집기의 현장노출시간을 단축하기 위한 방법으로 실행보정물질을 이용해 평형상태에서의 자유용존상 농도를 예측하는 방법이 가장 보편화되어 있으며, 해수의 경우 수동형채집기 표면에 난류를 형성시키거나 해류 흐름과 수평하게 설치함으로써 오염물질의 흡수속도를 증가시킬 수 있다. 퇴적물의 경우 현장에서의 시료를 실험실로 옮겨 슬러리로 만든 뒤 실험실 내에서 교반시키는 ex situ 방법을 사용한다.
국내에서도 현장노출시간을 단축시키는 다양한 방법들을 활용해 수동형채집기를 해양환경에 적용하게 된다면, 현재 분석법으로 측정하기 어려운 초저농도의 해수 및 충분한 시료확보가 어려운 퇴적물 공극수에서의 자유용존상 농도를 파악할 수 있다. 또한 퇴적물 환경관리를 위한 생물학적 영향 평가에 보다 정확한 화학 정보를 제시할 수 있을 것으로 판단된다.